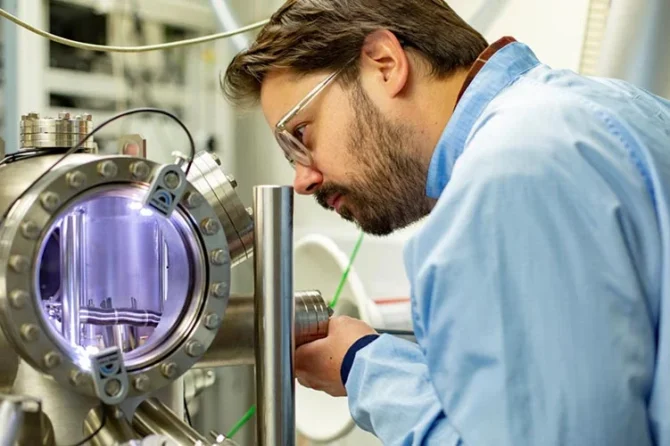
Chip Fabrication: Arsenic Atoms Transform Quantum Tech
UCL researchers have developed a groundbreaking technique using arsenic atoms in silicon that achieves a 97% success rate for single-atom placement (compared to phosphorus’s 70%), potentially solving quantum computing’s twin challenges of error rates and scalability through scanning tunneling microscopy hydrogen resist lithography.