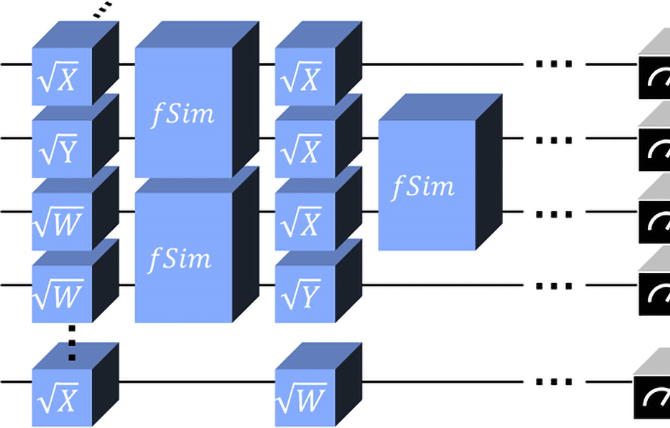
Classical Computing Overtakes Quantum Advantage: Google Sycamore Dethroned
Researchers successfully simulated Google’s 53-qubit Sycamore quantum circuit seven times faster than the original quantum computer using 1,432 GPUs and innovative tensor network algorithms, effectively refuting Google’s quantum advantage claim.